
Ski pole
Manon, hope for the 2030 Olympics ?
Our previous design of the ski pole aimed at Pierre-Luc, based on the principle of ball joint that enclosed his palm, does not seem appropriate to us in Manon’s case, because her clamp function can be performed by his thumb and his pinky. Of course the strength of this clamp is clearly not sufficient to hold a ski pole, so we keep the principle of using a socket build around her hand.
We therefore define new specifications intending the left hand palm to have the same feelings of touch as her right hand.
Scan of the left hand, equipped with a muffle
Pour ce nouveau projet, nous essayons de nous passer de l’étape moulage, en réalisant un scan de la main in-situ, en position de maintien du bâton de ski. La main étant équipée d’une moufle qui sera ensuite bien adaptée par la couturière de la famille.
For this new project, we are trying to work without the molding stage, by performing an in-situ live hand scan, right in the position of holding the ski pole. The hand being equipped with a muffle which will then be well adapted by her family’s seamstress (grand’ma).
The scanning operation is not as easy as expected, but after 3 attempts we got a good quality mesh file.

Once the STL is imported into an ONshape’s “part studio”, an enveloping surface is made around the mesh, made of multiple ‘Spline’ curves (generalization of Bezier curves) drawn over successive cut planes. These curves, building a group of sections, each of them wrapping the glove, are then connected (joined) to each other by ‘lofts’. A loft is a surface obtained by interpolation between the different curves, in the form of a NURBS surface (cf wikipedia).
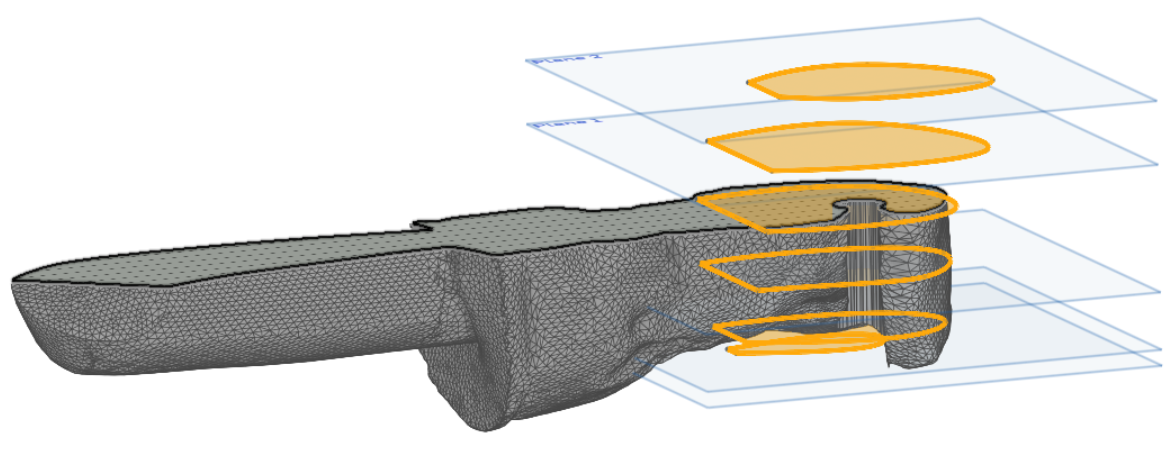


Highlights of the device including a (simple) system.
The new device is therefore no longer based on the ball joint principle but on a two-element system :
- an element on the stick (host)
- a detachable socket for Manon’s hand.
The cohesion of the two elements is achieved by neodymium magnets, powerful enough for the stick to follow each hand’s movements, but detachable enough to allow the release of the socket in case of fall.
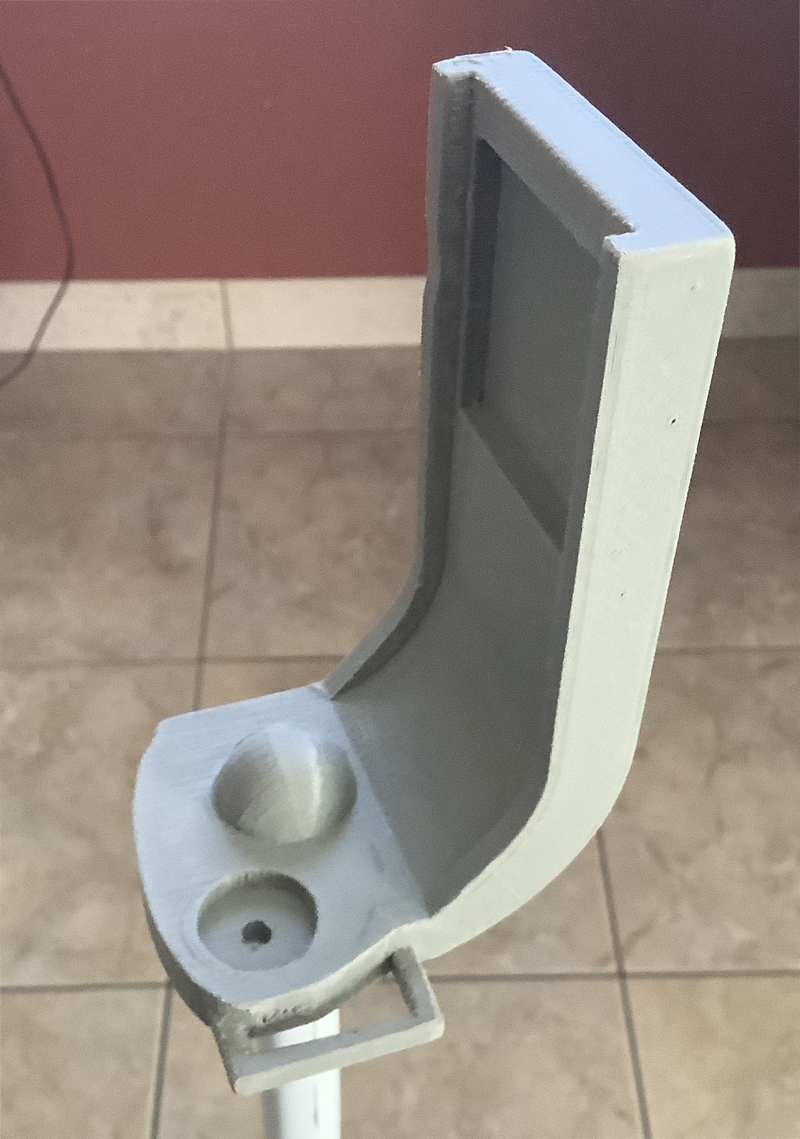
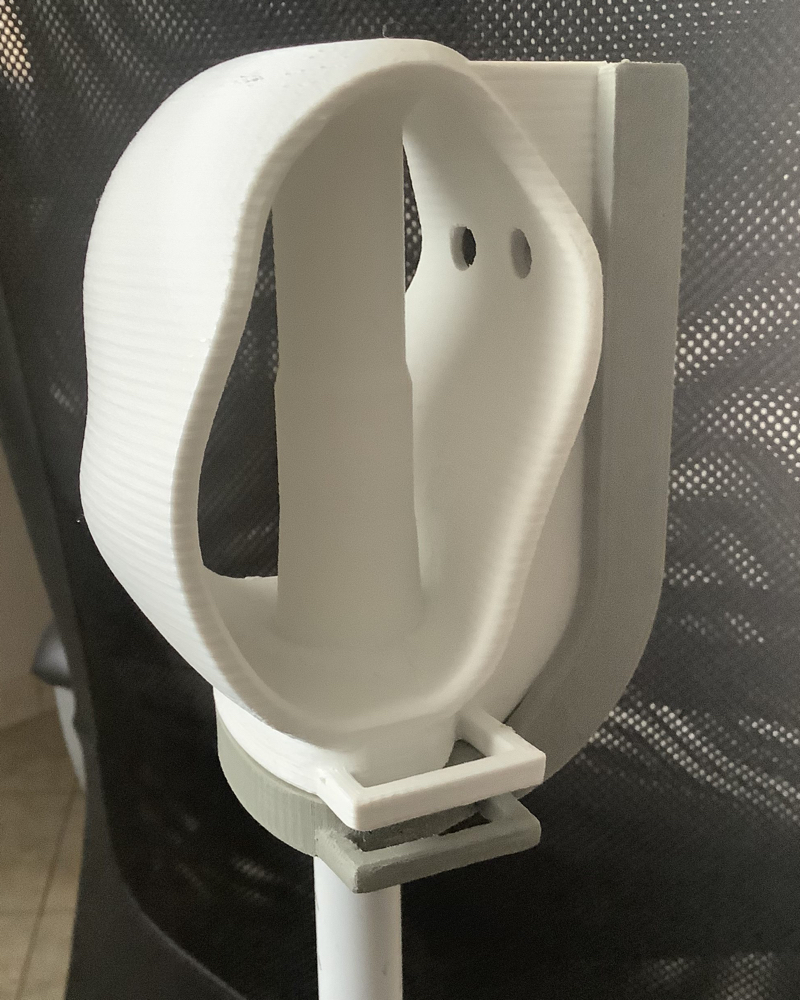
The hand-socket is “glued” to the host-frame thanks to the forces of attraction of the two magnets.
- The correct positioning of the interlocking is ensured by a centering dome, and a calibrated location
- The holding of the interlocking on the frame is the attractive forces of the main rectangular magnet (40x40x4)
- The second magnet (circular) facilitates vertical support and centering of the hand-socket.
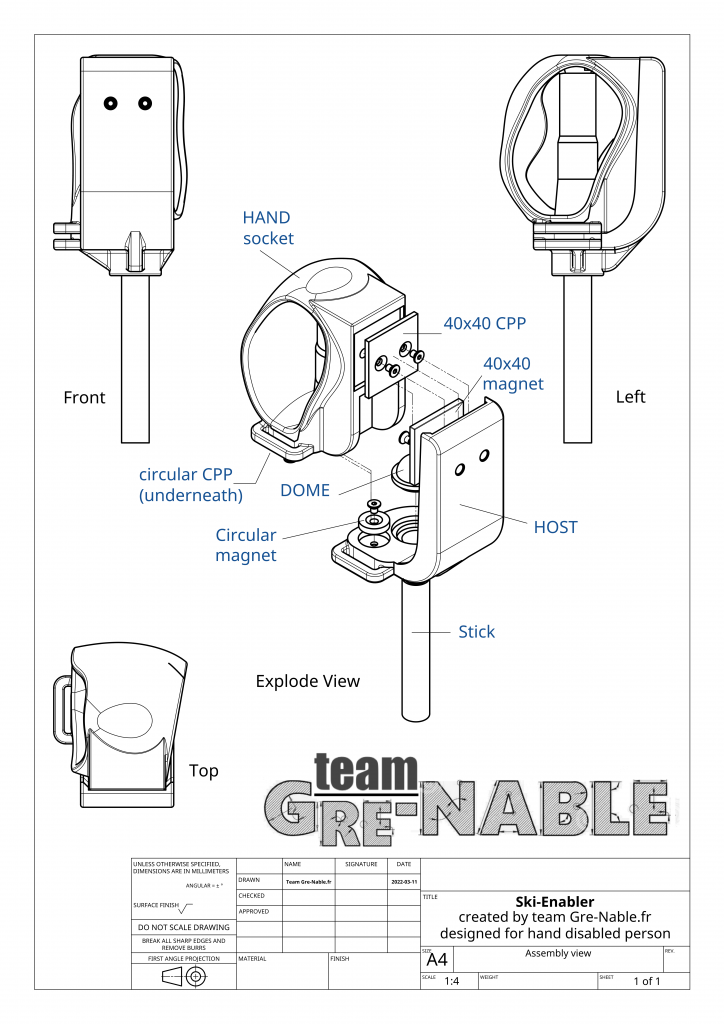
Some small improvements were made to give more room for the thumb and the final version of the interlocking was printed using semi-flexible material (BASF Fusion, with shore mark 65D).
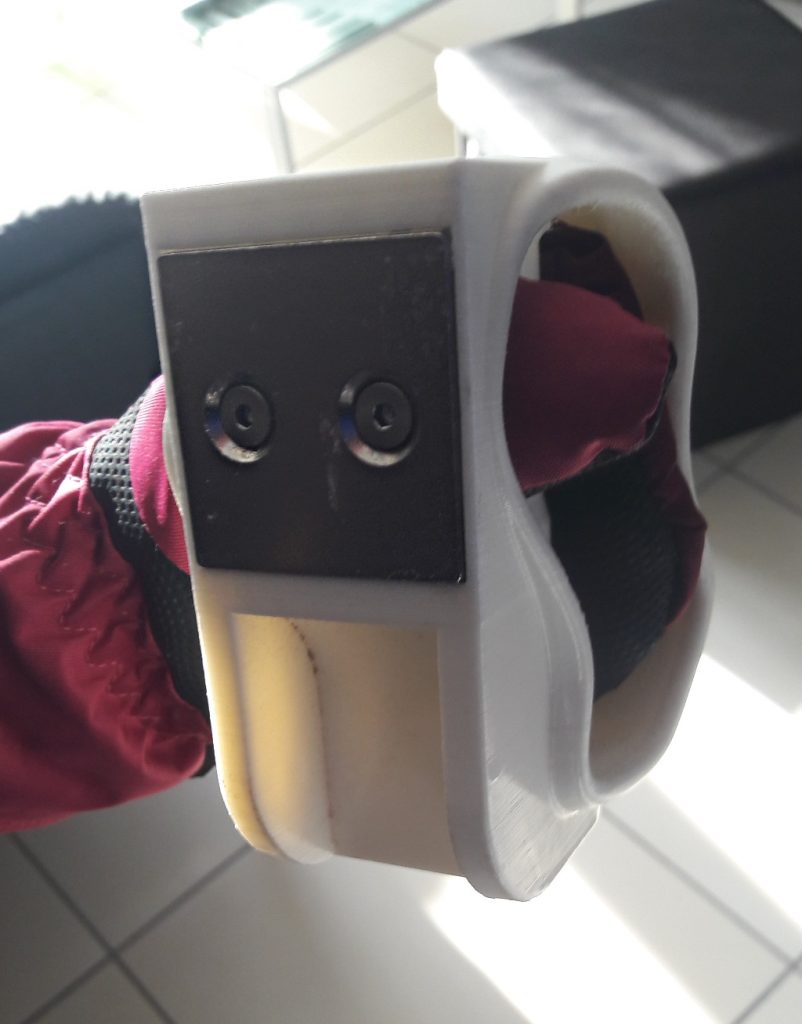

Ces adaptations de système à la main d’un autre enfant nécessitent de maitriser l’outil de conception CAO, mais n’est pas aussi compliqué qu’il y paraît. Les fichiers STL du système développé pour Manon ne seraient d’aucune utilité pour un autre enfant. Par contre, nos développements sont open-sources et disponibles sur la plate-forme Onshape, et nous sommes toujours prêts à donner un coup de main 🙂 [© E-nable France]
These system’s adaptations for another child’s hand will require some skill using a CAD design tool, but are not as complicated as it seems. The STL files of the system developed for Manon would be of no use for another child. On the other hand, our designs are open-source and available on the Onshape platform, and we are always ready to give a helpful hand 🙂
Let’s keep in touch.
Recent Comments